The hydrogenation of alkenes is a pivotal process that transforms unsaturated hydrocarbons into saturated ones. This process has vast applications in pharmaceuticals and petrochemicals.
Central to this transformative process is the role of catalysts, agents that expedite the reaction and influence its direction and efficiency. The performance of these catalysts underpins the success of hydrogenation, dictating both the quality and yield of the end product.
Continue reading as we explore the heart of catalyst functionality, dissecting how these substances are crucial in driving the efficiency and selectivity of hydrogenation processes.
Through this lens, we aim to uncover the nuanced interplay between catalyst composition, process conditions, and overall system design to enhance industrial hydrogenation practices.
Fundamentals of Alkene Hydrogenation
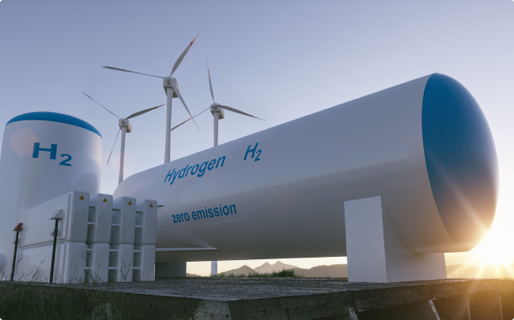
This fundamental transformation is not just a matter of adding hydrogen atoms to double bonds; it’s about harnessing the basic principles of chemistry to create molecules that form the backbone of numerous products and materials central to our daily lives and the economy.
At its core, alkene hydrogenation involves adding hydrogen (H₂) across the carbon-carbon double bond of alkenes, converting them into alkanes.
Catalysts, typically metals such as palladium, platinum, or nickel, facilitate this reaction by lowering the activation energy required for it to proceed.
The catalyst provides a surface upon which the reactants are adsorbed, aligning them to promote the efficient transfer of hydrogen atoms to the alkene, resulting in a saturated hydrocarbon molecule.
Significance in Creating Saturated Hydrocarbons
The creation of saturated hydrocarbons through hydrogenation is significant for several reasons.
- It alters the chemical and physical properties of the original molecule, influencing characteristics such as melting point, stability, and reactivity.
- Saturated hydrocarbons, or alkanes, are more versatile in their applications, serving as a key ingredient in fuels, and lubricants, and as raw materials in synthesizing plastics, pharmaceuticals, and other chemicals.
Types of Alkenes Commonly Subjected to Hydrogenation
The range of alkenes subjected to hydrogenation is broad, encompassing everything from simple molecules like ethylene and propylene to more complex structures found in natural oils and fats.
Each type of alkene brings its own set of challenges and opportunities for hydrogenation, influenced by factors like molecular size, the presence of substituents, and the degree of unsaturation.
Industrial Applications
The industrial applications of alkene hydrogenation are as varied as the types of alkenes themselves.
In the petrochemical industry, it’s a key step in refining processes, converting alkenes into alkanes for use in high-quality fuels. In the food industry, hydrogenation of vegetable oils creates semi-solid fats, a process crucial for producing margarine and shortening.
Meanwhile, in pharmaceuticals, hydrogenation is employed to synthesize a wide array of drug compounds, showcasing the versatility and indispensability of this reaction.
The hydrogenation of alkenes is more than just a chemical reaction; it’s a fundamental process that enables the transformation of raw materials into a multitude of products that support various sectors of the economy.
The choice of catalyst and understanding the nuances of the reaction are pivotal in optimizing the efficiency and outcomes of alkene hydrogenation, highlighting its central role in industrial chemistry.
Exploring Types of Catalysts For The Hydrogenation of Alkenes
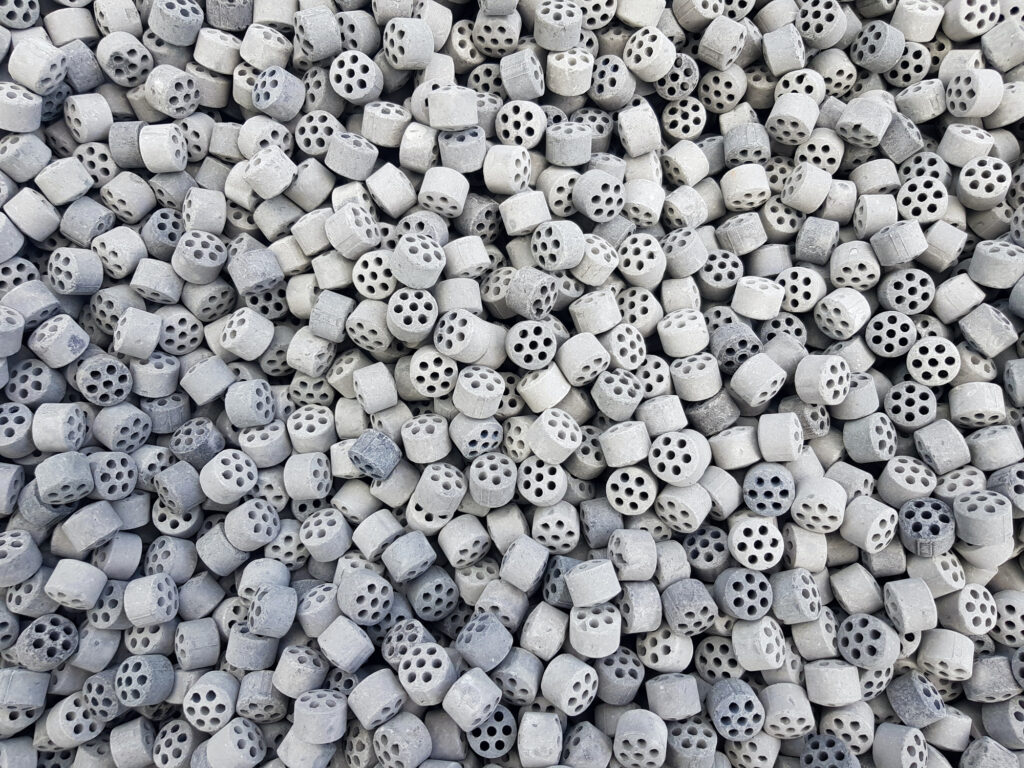
As previously mentioned, Hydrogenation is a cornerstone in various industrial applications, from food processing to fuel production.
This transformation’s effectiveness and efficiency heavily rely on the catalysts employed.
These catalysts can be broadly categorized into two types: heterogeneous and homogeneous.
Heterogeneous Catalysts
Heterogeneous catalysts are solid catalysts that exist in a different phase than the reactants, usually solid in a liquid or gas mixture. These catalysts facilitate the reaction at their surface.
Common heterogeneous catalysts include:
- Palladium (Pd): Offers high selectivity and activity, commonly supported on carbon or alumina.
- Nickel (Ni): Widely used due to its cost-effectiveness and robustness, suitable for large-scale industrial processes.
- Platinum (Pt): Known for its efficiency, although its higher cost limits its use to specialized applications.
Bi-metallics, Tri-metallics and Promoters that enhance selectivity:
Various mixtures of active metals may be used and metal oxide promoters may be added to improve the selectivity of the reaction to products with less by-products.
Performance Metrics
The effectiveness is often measured in terms of turnover frequency (the number of reactant molecules a catalyst site converts per unit time) and catalyst life (how long a catalyst maintains its activity).
Homogeneous Catalysts
Homogeneous catalysts are those where the catalyst is in the same phase (typically liquid) as the reactants. This uniformity allows for precise control over the reaction conditions and outcomes, which is particularly important in reactions requiring high specificity, such as producing enantiomerically pure compounds in the pharmaceutical industry.
The homogeneous nature of these catalysts facilitates intimate contact with reactants, enabling efficient catalysis and selective outcomes essential for creating active pharmaceutical ingredients (APIs) with the correct chiral orientation.
The use of ligands in these transition metal complexes to control reactivity and selectivity further emphasizes their role as homogeneous catalysts, allowing for fine-tuning catalyst properties to achieve desired reaction pathways and products.
Common homogeneous catalysts include:
Rhodium (Rh)
Rhodium complexes are renowned for their efficiency and selectivity in catalyzing the addition of hydrogen to double bonds, a critical step in creating chiral molecules.
Their ability to facilitate reactions under mild conditions while maintaining high selectivity for the desired enantiomer makes them invaluable in synthesizing active pharmaceutical ingredients (APIs), where the molecular orientation can significantly affect the drug’s efficacy and safety.
Ruthenium (Ru)
Like rhodium, ruthenium complexes also play a significant role in asymmetric hydrogenation.
Ruthenium catalysts are particularly appreciated for their robustness and versatility. They can catalyze a wide range of substrates, including those that are challenging for other catalysts.
Their application extends beyond pharmaceuticals, impacting the production of flavors, fragrances, and agrochemicals, where the precise control of molecular orientation is equally critical.
Advancements & Applications
The continued evolution of rhodium and ruthenium catalysts has broadened their applicability through innovations in ligand design and metal-ligand coordination chemistry.
These catalysts have enabled the scalable production of complex, chiral molecules with high purity and yield in pharmaceuticals. Their use ensures that medications are effective and meet the rigorous safety standards required by regulatory agencies.
Comparative Analysis
Palladium (Pd) & Platinum (Pt) | Nickel (Ni) | Homogeneous | Heterogeneous | |
Selectivity & Activity | Highly selective and active, making them ideal for fine chemical synthesis. | Less selective, preferred for bulk chemical processes due to robust performance. | High selectivity and activity, especially in reactions requiring precise control. | Varies; generally less selective than homogeneous catalysts. |
Cost | Higher cost justified by efficiency in producing high-value products. | Lower cost, attractive for large-scale operations. | Cost varies by metal and ligand; often higher due to the need for precision control. | Typically lower cost due to durability and reusability. |
Application Suitability | Suited for processes requiring high selectivity and activity, such as fine chemical synthesis. | Suited for large-scale, bulk chemical processes. | Preferred for reactions needing precise control over product stereochemistry. | Favored for their ease of separation and recyclability in continuous processes. |
Factors Affecting Catalyst Performance
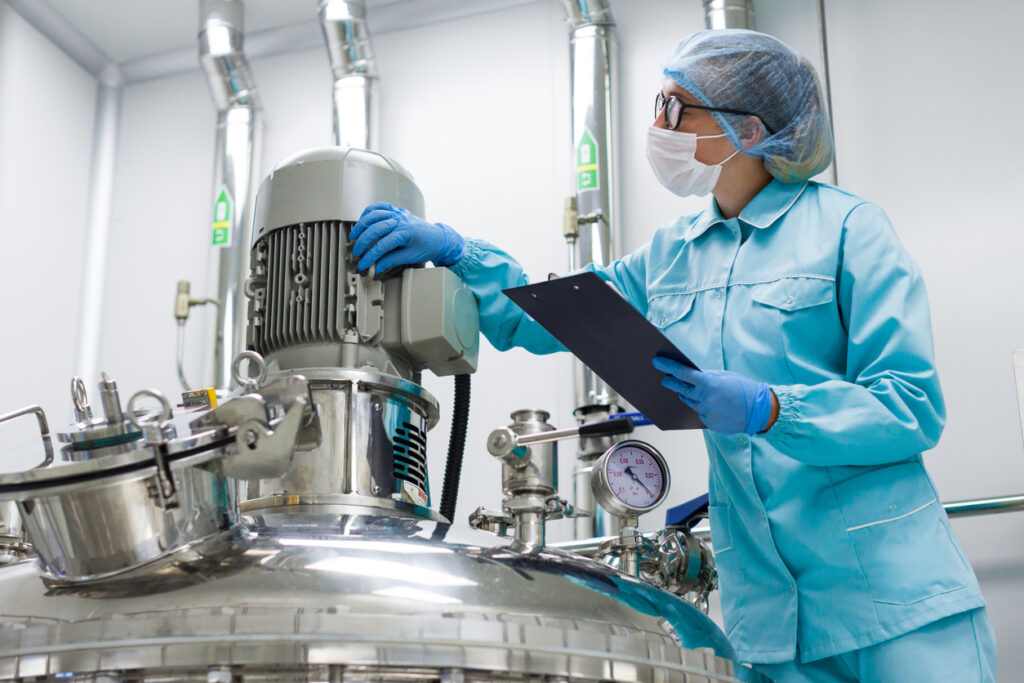
Understanding these factors and their interactions is essential for developing, selecting, and optimizing catalyst systems.
Controlling these variables makes it possible to tailor catalyst performance to specific industrial processes, achieving unparalleled efficiency and product quality.
Here are the primary factors that significantly impact catalyst performance:
Chemical Composition
- Catalyst Material: The inherent chemical properties of the catalyst material, including its electronic structure and surface chemistry, play a pivotal role in its activity and specificity.
- Ligands and Additives: In the case of homogeneous catalysts, such as rhodium and ruthenium complexes, ligands and other additives can modify the catalyst’s electronic environment, enhancing its reactivity and selectivity toward certain reactions.
Physical Properties
- Surface Area: For heterogeneous catalysts, a higher surface area provides more active sites for the reaction, directly impacting the catalyst’s effectiveness.
- Pore Size and Structure: The distribution of pore sizes and the overall structure can influence diffusion rates of reactants and products, affecting reaction efficiency and selectivity.
Reaction Conditions
- Temperature and Pressure: Optimal temperature and pressure conditions are critical for maximizing catalyst performance. Too high or too low can lead to decreased activity or unwanted side reactions.
- Atmosphere: The presence of gases, such as oxygen or hydrogen, can significantly affect the catalyst’s performance, potentially leading to oxidation or reduction of the catalyst material.
Catalyst Poisoning and Deactivation
- Poisoning: The presence of certain substances can bind to the catalyst’s active sites more strongly than the reactants, effectively “poisoning” the catalyst and reducing its activity.
- Sintering: High temperatures can cause catalyst particles to fuse, reducing the effective surface area and, consequently, the catalyst’s activity.
- Fouling: The accumulation of reaction by-products on the catalyst surface can block active sites, hindering the catalyst’s performance.
Operational Life
- Catalyst Stability: The structural stability of a catalyst under operational conditions determines its lifespan and the need for regeneration or replacement.
- Regeneration Capability: The ability to regenerate a catalyst’s active sites can significantly extend its operational life and enhance process economics.
Strategies for Optimizing Catalyst Performance
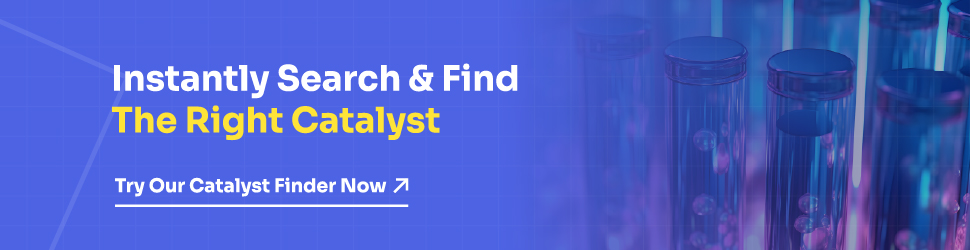
Each approach offers a way to tackle specific challenges associated with catalyst operation, from deactivation and poisoning to suboptimal reaction conditions.
Tailoring these strategies to the unique requirements of a given process ensures that catalysts deliver their full potential, driving efficiency, sustainability, and innovation in chemical manufacturing.
Catalyst Modification | |
Support Modification | Altering the support material of heterogeneous catalysts can improve dispersion and increase the available active surface area, enhancing catalytic activity. |
Metal Loading | Adjusting the amount of active metal on a catalyst can optimize its activity and selectivity for specific reactions. |
Promoters Addition | Incorporating small amounts of secondary components (promoters) can significantly enhance the catalyst’s activity and stability. |
Optimal Process Conditions | |
Temperature & Pressure Optimization | Fine-tuning reaction temperatures and pressures can help reach the optimal balance between reaction rate and desired product formation. |
Gas Flow Rate Adjustments | In gas-phase reactions, optimizing the flow rate of reactants can improve contact efficiency between the reactants and the catalyst, leading to better conversion rates. |
Use of Additives | |
Poison Inhibitors | Adding chemicals that selectively bind to poisons or impurities in the feedstock can protect the catalyst and prolong its life. |
Dispersants | Utilizing dispersants can prevent the aggregation of catalyst particles, maintaining a high surface area for reactions. |
Regeneration Techniques | |
Thermal Treatment | Periodic heating of the catalyst in an appropriate atmosphere can burn off accumulated deposits, restoring the catalyst’s activity. |
Chemical Regeneration | Treating the catalyst with specific chemicals can remove poisons or regenerate active sites, enhancing performance. |
Advanced Manufacturing Techniques | |
Nanostructuring | Developing catalysts at the nanoscale can significantly increase their surface area-to-volume ratio, improving their activity and selectivity. |
Molecular Engineering | Designing catalysts at the molecular level, particularly for homogeneous catalysts, can allow for unprecedented control over reaction mechanisms and pathways. |
Implementation of Real-Time Monitoring | |
Spectroscopic Techniques | Employing spectroscopic methods to monitor the catalyst’s state during reaction can provide immediate feedback for adjusting process conditions to maintain optimal performance. |
Computational Modeling | Using computational tools to model catalyst behavior under different conditions can predict optimal operation parameters without extensive trial and error. |
By employing these strategies, manufacturers and researchers can significantly improve the performance of catalysts used in various industrial applications.
Choosing the Right Catalyst for Your Process

When considering which hydrogenation catalyst best suits your needs, it’s crucial to evaluate several key factors.
- Process Requirements: Understanding the specifics of your process, including reaction conditions and the nature of the reactants and desired products, will help identify the catalyst that offers the optimal activity and selectivity.
- Product Specifications: The desired properties of your final product, especially in terms of purity and isomer distribution, can significantly influence catalyst choice.
- Economic Factors: Beyond performance, the cost, availability, and reusability of a catalyst are essential considerations. An effective catalyst that reduces the need for frequent replacement or regeneration can offer substantial long-term savings.
Selecting the most suitable catalyst for your alkene hydrogenation process is not just a matter of scientific precision; it’s a strategic decision that directly impacts your operation’s efficiency, product quality, and economic viability.
The challenge often lies in pinpointing the one that aligns perfectly with your process requirements and product specifications.
This is where Applied Catalyst comes into play, serving as a pivotal resource in guiding you through the catalyst selection, production, and optimization maze.
Partner With Applied Catalysts
Applied Catalyst stands at the forefront of catalyst technology, offering unparalleled expertise and resources to assist in selecting, developing, and optimizing the perfect catalyst for your hydrogenation process. Our approach combines:
- Custom Catalyst Development: Tailoring catalysts to meet the exact needs of your process, ensuring maximum efficiency and product quality.
- Economic Viability: Balancing high performance with cost-effectiveness, we focus on solutions that enhance the overall process economy, considering factors like catalyst lifespan and the potential for regeneration.
- Optimization Services: Beyond initial selection, our team provides ongoing support to refine and enhance catalyst performance, adapting to any changes in your process requirements.
The right catalyst can transform your operations, yielding higher efficiencies, superior product quality, and better economic outcomes. Our expertise and custom solutions ensure that your catalyst meets and exceeds expectations, propelling your processes to new heights of success.
Get in touch with our team today and request a free quote tailored to your process needs and requirements.
Recent Posts
Manufacturing Compliance: Monitor and Reduce VOC Emissions Effectively
As companies strive to meet environmental standards, VOC (Volatile Organic Compounds) abatement is a critical focus area, particularly for the manufacturing industry. Staying compliant with local and national regulations is…
Read MoreUnderstanding Catalyst Deactivation: How Characterization Can Identify the Root Cause
AbstractCatalyst deactivation is a common challenge in many catalytic processes, but by identifying the root causes, taking appropriate corrective actions, and utilizing advanced characterization techniques, it is possible to maintain…
Read MoreChallenges in Catalyst Regeneration and How to Overcome Them
Learn the techniques for effective catalyst regeneration and how it can optimize your operations. Read now to enhance your industrial processes!
Read More