Abstract Catalyst deactivation is a common challenge in many catalytic processes, but by identifying the root causes, taking appropriate corrective actions, and utilizing advanced characterization techniques, it is possible to maintain and even enhance catalyst performance. Proper characterization helps resolve current issues and provides insights that can lead to significant efficiency gains and cost savings. |
Catalyst deactivation is a significant concern in catalytic processes, impacting both performance and efficiency. When catalysts lose their activity, it can lead to increased emissions, higher operational costs, and potential downtime. While deactivation is inevitable, it can be slowed or prevented by taking a systematic monitoring approach.
Understanding and identifying the root cause of catalyst deactivation is the primary way to maintain optimal catalytic performance and ensure that processes remain efficient and compliant with environmental standards. This blog will explore catalyst deactivation causes, methods to identify them, and how proper characterization can enhance overall process efficiency.
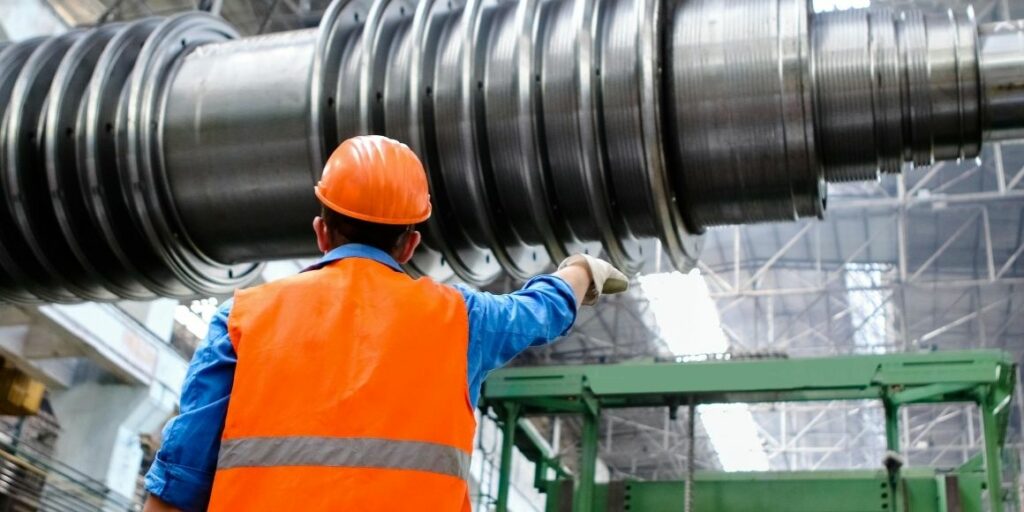
Identifying Catalyst Deactivation Causes
Catalyst deactivation refers to the gradual decline in catalyst activity, leading to reduced efficiency in chemical reactions. Deactivation mechanisms fall into three main categories: chemical, mechanical, and thermal. In this section, we will review common deactivation causes and the broad solution. In the following sections, we will examine these solutions in depth.
1. Chemical Deactivation
Catalyst Poisoning: This occurs when impurities in the exhaust stream bind strongly to active sites on the catalyst surface, making these sites unavailable for the intended reaction. Poisoning can be fast or slow and reversible or irreversible, depending on the nature and concentration of the poison.
Common poisons include silicon, sulfur, arsenic, and coke. To mitigate poisoning, it is crucial to purify the reactant streams and use appropriate guard beds.
Vapor and Solid Reactions: Catalysts can undergo chemical reactions with vapors or solids, forming inactive compounds or volatile substances that exit the reactor.
How To Manage: These reactions can be managed by controlling reaction conditions and choosing a catalyst design less susceptible to such transformations.
2. Mechanical Deactivation:
Fouling / Masking: This mechanism involves the deposition of elemental species and compounds from the exhaust stream onto the catalyst surface, blocking active sites and pores.
Masking is particularly problematic when organic bound Silicon or Phosphorus decomposes either directly on the catalyst surface or upstream of the catalyst bed.
How To Manage: A condition and catalyst assessment is needed to understand if another catalyst or guard bed is needed or if the species introducing potential masking agents can be eliminated from the exhaust stream to prolong the catalyst’s life.
Attrition and Crushing: Catalysts can break down due to mechanical stresses such as collisions between particles or with reactor walls, especially in fluidized or slurry beds. This degradation can also be caused by thermal or chemical stresses.
How To Manage: Enhancing catalyst strength through improved preparation methods and adding binders can help mitigate attrition and crushing.
3. Thermal Deactivation:
Sintering: High temperatures can cause catalyst particles to agglomerate, reducing surface area and catalytic activity. This process, known as sintering, is accelerated by the presence of water vapor and is irreversible.
How To Manage: Sintering can be minimized by operating at lower temperatures, perhaps adding dilution air to limit exothermic reactions and using catalyst formulations that resist precious metal particle agglomeration.
Methods for Identifying Causes
To effectively address catalyst deactivation, it is crucial to identify the root cause. Catalyst characterization plays a vital role in this process, offering insights into the physical and chemical changes that occur during deactivation.
Techniques such as BET surface area analysis, elemental analysis, spectroscopy, and temperature-programmed methods are instrumental in identifying issues like catalytic site blockage or loss of active sites.
For instance, BET surface area analysis can reveal reductions in the catalyst’s active surface area, indicating thermal degradation or fouling.
Elemental analysis, such as XRF (X-ray fluorescence spectroscopy) and PIXE (Proton induced X-ray emission) can identify foreign matter that has been deposited onto the catalyst surface, blocking catalytic activity.
Spectroscopy techniques, such as X-ray photoelectron spectroscopy (XPS), can detect the presence of poisons on the catalyst’s surface, providing clues about contamination.
Temperature-programmed desorption (TPD) can help determine the strength of adsorption of different species on the catalyst, offering insights into potential poisoning or fouling mechanisms.
A root cause analysis is no small task, and both industrial plants and machinery suppliers often struggle with the time and equipment needed to make a precise diagnostic. That’s why outsourcing this service is usually recommended, keeping in mind that it should be a properly equipped and experienced partner.
Choosing The Right Partner
Applied Catalysts operates a fully equipped analytical laboratory capable of diagnosing deactivation causes. Through detailed catalyst testing and cleaning procedures, we assess whether a deactivated catalyst can be restored to its original performance or if replacement should be considered.
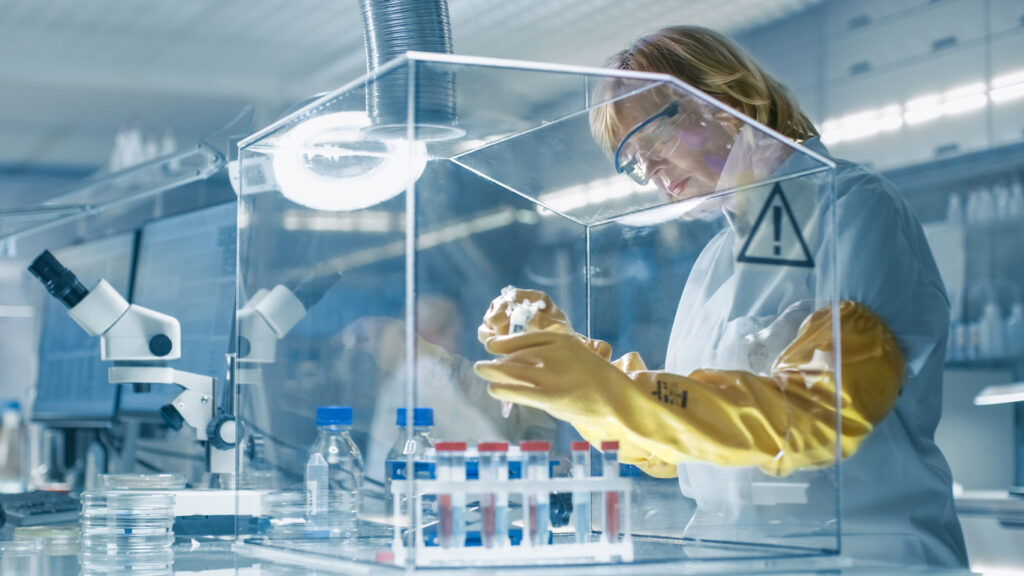
Optimizing Catalyst Performance After Deactivation is Detected
Actions to Take
Once deactivation is detected, several actions can be taken to restore catalyst performance. Depending on the severity and type of deactivation, the catalyst may require cleaning, regeneration, or complete replacement. Cleaning involves removing contaminants or deposits from the catalyst surface, often through chemical or thermal treatments. Regeneration typically involves oxidizing or reducing the catalyst to restore its active sites.
In addition to these direct actions, modifying operating conditions can mitigate further deactivation. For example, adjusting the reaction temperature, pressure, or feed composition (ex: potential dilution air addition) can help prevent thermal deactivation or reduce the impact of poisons. Implementing preventative measures, such as feedstock purification or catalyst protection layers, can extend the catalyst’s life and maintain optimal performance.
What Happens If I Need A New Catalyst?
If you need to replace your catalyst, you’ll want to choose a suitable catalyst manufacturer. Here are a few tips on how to select a provider:
- Ensure the quality of the catalyst meets evolving industry standards, which can impact process efficiency and sustainability.
- Consider the manufacturer’s technical knowledge, track record, and ability to customize solutions for your specific needs.
- Assess their research capabilities, quality assurance, and regulatory compliance.
- Evaluate their post-purchase support and transparency in communication by checking out reviews.
- Finally, review their approach to sustainability, ethical manufacturing, and cost-effectiveness.
Improving Process Efficiency Through Catalyst Characterization
Proper catalyst characterization helps identify deactivation causes and provides actionable insights that can significantly improve process efficiency.
Applied Catalysts leverages advanced characterization techniques to offer tailored solutions that address specific deactivation issues. For example, by analyzing catalytic surface area and activity, we can recommend adjustments in reaction conditions or suggest alternative catalyst formulations to improve performance.
Studies show that effective catalyst monitoring and maintenance can enhance catalyst lifespans by up to 50% and reduce unplanned production shutdowns by as much as 10%.
Let Us Catalyze Your Business
Ongoing catalyst maintenance and characterization are essential for sustaining efficient, compliant, and cost-effective catalytic processes. By proactively addressing deactivation issues, you can ensure that your operations continue to perform at their best.
At Applied Catalysts, we offer various services in catalyst characterization and process optimization. Our team of experts is adept at identifying and resolving deactivation issues, ensuring that your catalytic processes remain efficient and cost-effective. Whether you need routine monitoring, in-depth analysis, or customized solutions, Applied Catalysts is your partner in maintaining optimal catalyst performance.
Contact Applied Catalysts today for expert advice and comprehensive catalyst characterization and deactivation prevention services.
Recent Posts
Manufacturing Compliance: Monitor and Reduce VOC Emissions Effectively
As companies strive to meet environmental standards, VOC (Volatile Organic Compounds) abatement is a critical focus area, particularly for the manufacturing industry. Staying compliant with local and national regulations is…
Read MoreChallenges in Catalyst Regeneration and How to Overcome Them
Learn the techniques for effective catalyst regeneration and how it can optimize your operations. Read now to enhance your industrial processes!
Read MoreRegenerative Thermal Oxidizer (RTO) to RCO Conversion
Regenerative thermal oxidizers (RTOs) are a leading solution for controlling volatile organic compounds (VOCs) and hazardous air pollutants (HAPs) from industrial air pollution sources. These systems can achieve up to…
Read More